defination
Electrochemistry is the study of chemical processes that cause electrons to move. This movement of electrons is called electricity, which can be generated by movements of electrons from one element to another in a reaction known as an oxidation-reduction ("redox") reaction
or
we can say that electrochemistry is the branch of science in which we study about the energy relationship between chemical energy and electrical energy produced during redox
voltic cell
reactionElectrochemical cells that use an oxidation-reduction reaction to generate an electric current are known as galvanic or voltaic cells. Because the potential of these cells to do work by driving an electric current through a wire is measured in units of volts, we will refer to the cells that generate this potential from now on as voltaic cells.
Within each half-cell, reaction occurs on the surface of the metal electrode. At the zinc electrode, zinc atoms are oxidized to form Zn2+ ions, which go into solution. The electrons liberated in this reaction flow through the zinc metal until they reach the wire that connects the zinc electrode to the platinum wire. They then flow through the platinum wire, where they eventually reduce an H+ ion in the neighboring solution to a hydrogen atom, which combines with another hydrogen atom to form an H2 molecule.
Electrochemistry is the study of chemical processes that cause electrons to move. This movement of electrons is called electricity, which can be generated by movements of electrons from one element to another in a reaction known as an oxidation-reduction ("redox") reaction
or
we can say that electrochemistry is the branch of science in which we study about the energy relationship between chemical energy and electrical energy produced during redox
voltic cell
reactionElectrochemical cells that use an oxidation-reduction reaction to generate an electric current are known as galvanic or voltaic cells. Because the potential of these cells to do work by driving an electric current through a wire is measured in units of volts, we will refer to the cells that generate this potential from now on as voltaic cells.
Let's take another look at the voltaic cell in the figure below.
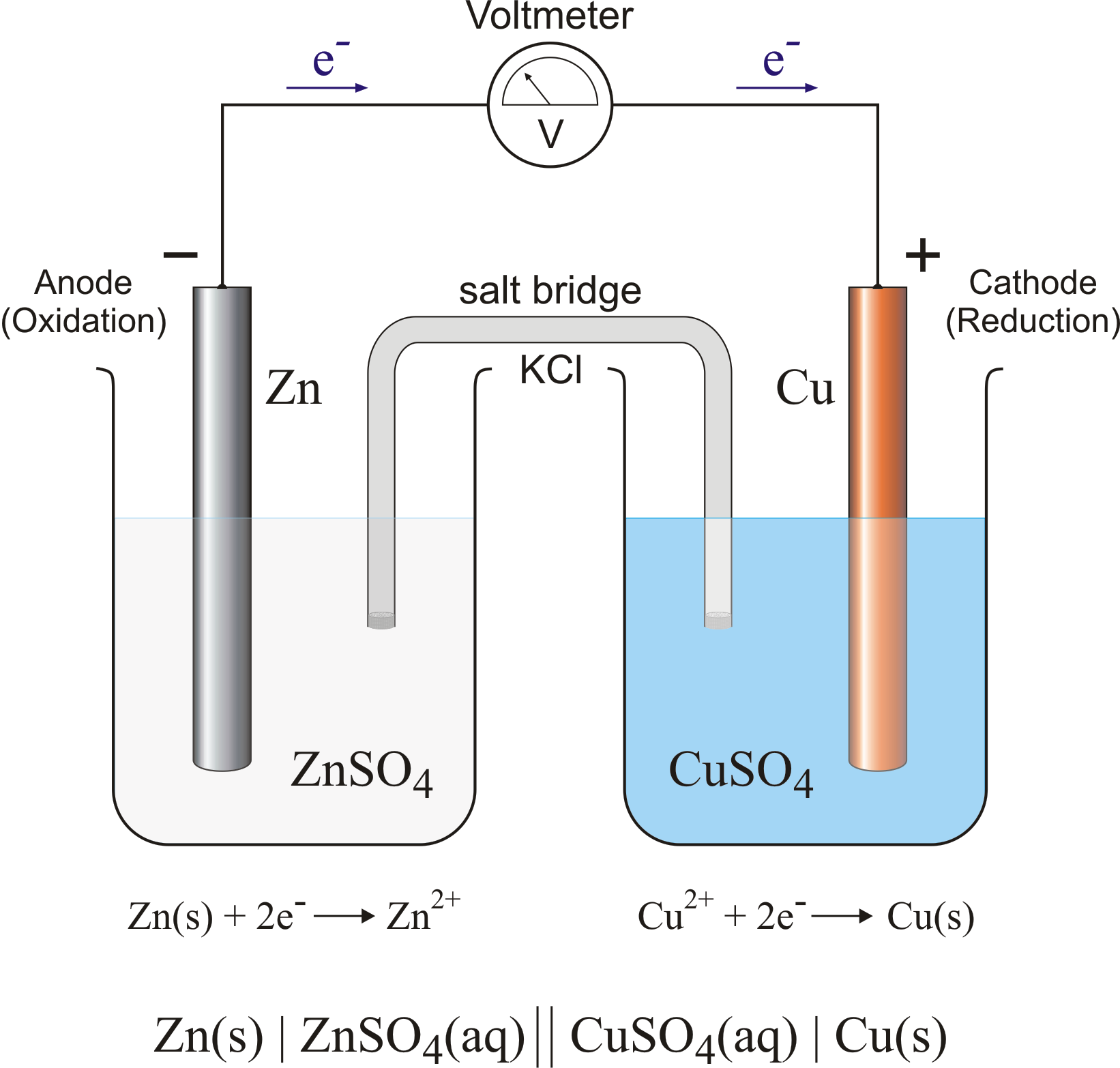
The electrode at which oxidation takes place in a electrochemical cell is called the anode. The electrode at which reduction occurs is called the cathode. The identity of the cathode and anode can be remembered by recognizing that positive ions, or cations, flow toward the cathode, while negative ions, or anions, flow toward the anode. In the voltaic cell shown above, H+ ions flow toward the cathode, where they are reduced to H2 gas. On the other side of the cell, Cl- ions are released from the salt bridge and flow toward the anode, where the zinc metal is oxidized.
The cell potential for a voltaic cell is literally the potential of the cell to do work on its surroundings by driving an electric current through a wire. By definition, one joule of energy is produced when one coulomb of electrical charge is transported across a potential of one volt.
The potential of a voltaic cell depends on the concentrations of any species present in solution, the partial pressures of any gases involved in the reaction, and the temperature at which the reaction is run. To provide a basis for comparing the results of one experiment with another, the following set of standard-state conditions for electrochemical measurements has been defined.
- All solutions are 1 M.
- All gases have a partial pressure of 0.1 MPa (0.9869 atm).
Although standard-state measurements can be made at any temperature, they are often taken at 25oC.
Cell potentials measured under standard-state conditions are represented by the symbol Eo. The standard-state cell potential, Eo, measures the strength of the driving force behind the chemical reaction. The larger the difference between the oxidizing and reducing strengths of the reactants and products, the larger the cell potential. To obtain a relatively large cell potential, we have to react a strong reducing agent with a strong oxidizing agent.
Example: The experimental value for the standard-state cell potential for the reaction between zinc metal and acid is 0.76 volts.
Zn(s) + 2 H+(aq) =Zn2+(aq) + H2(g) | Eo = 0.76 V |
The cell potential for this reaction measures the relative reducing power of zinc metal compared with hydrogen gas. But it doesn't tell us anything about the absolute value of the reducing power for either zinc metal or H2.
We therefore arbitrarily define the standard-state potential for the reduction of H+ ions to H2 gas as exactly zero volts.
2 H+ + 2 e- =H2 | Eo = 0.000... V |
We will then use this reference point to calibrate the potential of any other half-reaction.
The key to using this reference point is recognizing that the overall cell potential for a reaction must be the sum of the potentials for the oxidation and reduction half-reactions.
Eooverall = Eoox + Eored
If the overall potential for the reaction between zinc and acid is 0.76 volts, and the half-cell potential for the reduction of H+ ions is 0 volts, then the half-cell potential for the oxidation of zinc metal must be 0.76 volts.
Zn = Zn2+ + | Eoox = 0.76 V |
+ 2 H+ + ------------------------ | Eored = 0.00 V ------------------- |
Zn + 2 H+ = Zn2+ + H2 | Eo = Eoox + Eored = 0.76 V |
Predicting Spontaneous Redox Reactions From the Sign of Eo
The magnitude of the cell potential is a measure of the driving force behind a reaction. The larger the value of the cell potential, the further the reaction is from equilibrium. The sign of the cell potential tells us the direction in which the reaction must shift to reach equilibrium.
Consider the reaction between zinc and acid, for example.
Zn(s) + 2 H+(aq) = Zn2+(aq) + H2(g) | Eo = 0.76 V |
The fact that Eo is positive tells us that when this system is present at standard-state conditions, it has to shift to the right to reach equilibrium. Reactions for which Eo is positive therefore have equilibrium constants that favor the products of the reaction. It is tempting to describe these reactions as "spontaneous."
What happens to the cell potential when we reverse the direction in which a reaction is written? Turning the reaction around doesn't change the relative strengths of the oxidizing or reducing agents. The magnitude of the potential must remain the same. But turning the equation around changes the sign of the cell potential, and can therefore turn an unfavorable reaction into one that is spontaneous, or vice versa.
Standard-State Reduction Half-Cell Potentials
The standard-state cell potentials for some common half-reactions are given in the table below.
Standard-State Reduction Potentials,
Half-Reaction | Eored | |||||
K+ + e- =K | -2.924 | Best | ||||
Ba2+ + 2 e- = Ba | -2.90 | reducing | ||||
Ca2+ + 2 e- = Ca | -2.76 | agents | ||||
Na+ + e- = Na | -2.7109 | |||||
Mg2+ + 2 e- = Mg | -2.375 | |||||
H2 + 2 e- = 2 H- | -2.23 | |||||
Al3+ + 3 e- = Al | -1.706 | |||||
Mn2+ + 2 e- = Mn | -1.04 | |||||
Zn2+ + 2 e- = Zn | -0.7628 | |||||
Cr3+ + 3 e- = Cr | -0.74 | |||||
S + 2 e- = S2- | -0.508 | |||||
2 CO2 + 2 H+ + 2 e- = H2C2O4 | -0.49 | |||||
Cr3+ + e- = Cr2+ | -0.41 | |||||
Fe2+ + 2 e- = Fe | -0.409 | |||||
Co2+ + 2 e- = Co | -0.28 | |||||
Ni2+ + 2 e- = Ni | -0.23 | |||||
Sn2+ + 2 e- = Sn | -0.1364 | |||||
Pb2+ + 2 e- = Pb | -0.1263 | |||||
Fe3+ + 3 e- = Fe | -0.036 | |||||
2 H+ + 2 e- = H2 | 0.0000... | |||||
S4O62- + 2 e- = 2 S2O32- | 0.0895 | |||||
Oxidizing | Sn4+ + 2 e- = Sn2+ | 0.15 | ![]() | |||
power | Cu2+ + e- = Cu+ | 0.158 | Reducing | |||
increases | Cu2+ + 2 e- = Cu | 0.3402 | power | |||
O2 + 2 H2O + 4 e- = 4 OH- | 0.401 | increases | ||||
Cu+ + e- = Cu | 0.522 | |||||
I3- + 2 e- = 3 I- | 0.5338 | |||||
MnO4- + 2 H2O + 3 e- = MnO2 + 4 OH- | 0.588 | |||||
O2 + 2 H+ + 2 e- = H2O2 | 0.682 | |||||
Fe3+ + e- = Fe2+ | 0.770 | |||||
Hg22+ + 2 e- = Hg | 0.7961 | |||||
Ag+ + e- = Ag | 0.7996 | |||||
Hg2+ + 2 e- = Hg | 0.851 | |||||
H2O2 + 2 e- = 2 OH- | 0.88 | |||||
HNO3 + 3 H+ + 3 e- = NO + 2 H2O | 0.96 | |||||
Br2(aq) + 2 e- = 2 Br- | 1.087 | |||||
1.19 | ||||||
CrO42- + 8 H+ + 3 e- =Cr3+ + 4 H2O | 1.195 | |||||
Pt2+ + 2 e- = Pt | 1.2 | |||||
MnO2 + 4 H+ + 2 e- = Mn2+ + 2 H2O | 1.208 | |||||
O2 + 4 H+ + 4 e- = 2 H2O | 1.229 | |||||
Cr2O72- + 14 H+ + 6 e- = 2 Cr3+ + 7 H2O | 1.33 | |||||
Cl2(g) + 2 e- = 2 Cl- | 1.3583 | |||||
PbO2 + 4 H+ + 2 e- = Pb2+ + 2 H2O | 1.467 | |||||
MnO4- + 8 H+ + 5 e- = Mn2+ + 4 H2O | 1.491 | |||||
Au+ + e- = Au | 1.68 | |||||
H2O2 + 2 H+ + 2 e- =2 H2O | 1.776 | |||||
Co3+ + e-2 =Co2+ | 1.842 | |||||
Best | S2O82- + 2 e- = 2 SO42- | 2.05 | ||||
oxidizing | O3(g) + 2 H+ + 2 e- = O2(g) + H2O | 2.07 | ||||
agents | F2(g) + 2 H+ + 2 e- = 2 HF(aq) | 3.03 |
Balancing Redox Reactions
Method 1: Oxidation Number Method
- Step 1: Assign oxidation numbers to each atom.
- Step 2: Determine the net change in charge to determine the ratio of atoms
- Step 3: Use the ratio to eliminate the net charge change
- Step 4: Use the ratio as coefficients for the elements
- Step 5: Add H+ (under acidic conditions), OH- (under basic conditions), and H2O to balance charges.
Method 2: Half-Reaction Method
- Step 1: Determine oxidation numbers for each atom
- Step 2: Use oxidation numbers to determine what is oxidized and what is reduced.
- Step 3: Write a half-reaction for reduction
- Step 4: Write a half-reaction for oxidation
- Step 5: Balance all elements except H and O
- if have acid redox reaction: Balance the O using , balance the using protons
- if have base redox reaction: Balance O using
- Step 6: Add up the charge on each side
- Step 7: Balance the charges by adding electrons
- Step 8: Multiply the half-reactions by factors that cancel out electrons
- Step 9: Add the two half-reactions back together to eliminate out intermediate
- Free elements have an oxidation state of 0. (e.g., He, N2, O2 has an oxidation state of 0)
- The oxidation state of one atom ion must equal the net charge. ( Ex: F- oxidation state is -1, K+ oxidation state is +1)
- The sum of the oxidation state has to equal the total net charge for a compound. (Ex: MnO4 has a net charge of -1, Mn(+7)O4(-8)= -1)
- The alkali metals (Group I elements) have an oxidation state of +1. (EX: Li2O, Li= +1)
- The alkaline earth metals (Group II elements) always have an oxidation state of +2. (Ex: CaO, Ca=+2)
- Oxygen has an oxidation state of -2 in a compound
- Fluorine has an oxidation state of -1 in a compound
- Hydrogen has an oxidation state of +1 in a compound.
- Transition metals and other metals may have more than one common ionic charge. ( EX: Chromium's common ionic charges are Cr+2 and Cr+3
Terminology
- Anode: Electrode in an electrochemical cell on which the oxidation reaction occurs.
Cathode: Electrode in an electrochemical cell on which the reduction reaction occurs<iframe style="width:120px;height:240px;" marginwidth="0" marginheight="0" scrolling="no" frameborder="0" src="//ws-in.amazon-adsystem.com/widgets/q?ServiceVersion=20070822&OneJS=1&Operation=GetAdHtml&MarketPlace=IN&source=ac&ref=qf_sp_asin_til&ad_type=product_link&tracking_id=technologyi06-21&marketplace=amazon®ion=IN&placement=B013I2YJTO&asins=B013I2YJTO&linkId=4951db99a0056fb35f36f4b413e05900&show_border=false&link_opens_in_new_window=false&price_color=333333&title_color=0066C0&bg_color=FFFFFF"> </iframe>
No comments:
Post a Comment